Suitability of denervated muscle flaps as recipient sites for pancreatic islet cell transplantation
Article information
Abstract
Background
Extensive research has been conducted on islet transplantation as a possible cure for diabetes. Islet transplantation in the liver via the portal vein has shown remarkable results, but numerous other recipient sites are currently being investigated. We aimed to show the effectiveness of using a muscle flap as a recipient site for islet transplantation.
Methods
Islet cells were harvested from 12 isogenic Lewis rats, and then diabetes was induced in another 12 isogenic Lewis rats by streptozotocin injection. In six rats, 3,000 islets were transplanted into gastrocnemius muscle flaps, and in the other six rats, the same number of islets were transplanted into the gastrocnemius muscle. The transplanted islet cell function between the two groups was compared by means of blood glucose tests, glucose tolerance tests, immunohistochemistry, and real-time reverse transcription polymerase chain reaction.
Results
In the muscle flap group, blood glucose levels significantly decreased after islet transplantation. Blood glucose levels were significantly different between the two groups at 3 weeks after transplantation. The muscle flap group showed nearly normoglycemic results upon the glucose tolerance test, whereas the muscle group was hyperglycemic. Immunohistochemical evaluation showed positive results against insulin and glucagon in biopsies of both groups, and the islet cell density was higher in the muscle flap group. There were no statistically significant differences between the two groups in real-time reverse transcription polymerase chain reaction results.
Conclusions
Our results suggest that muscle flaps are promising candidates for islet cell transplantation.
INTRODUCTION
In type 1 diabetes patients, pancreatic islet cell transplantation is an epoch-making therapy. According to the International Islet Transplantation Registry, most clinical transplants have been performed in the liver through the portal vein. However, transplantation into the hepatic parenchyma is time-consuming and often requires re-transplantation because a large number of islet cells can be lost during the injection and in the early engraftment stage [1]. Studies have highlighted that oxygen partial pressure is lower in the liver than in the pancreas [2]. Moreover, immune responses and toxic effects derived from the digestive system were reported [3]. Transplantation into the kidney capsule has significant disadvantages in the anatomical approach when applied to large animals or humans.
Therefore, studies have reported the requirements for appropriate recipient sites. Pancreatic islet cells need sufficient oxygen and glucose, an appropriate pH level, and an environment with minimal toxic radicals [4]. For vascularization, proximity to blood vessels is essential. Furthermore, limited space and high vascular distribution are recommended, as the cell size is usually approximately 50–100 μm. Adequate communication pathways are also required to control insulin secretion in response to blood glucose levels [5]. Muscles are known to have abundant blood distribution and excellent accessibility. Several studies have argued that striated muscle is a promising site for islet cell transplantation [6-9]. However, the contractile motion of the muscle may decrease blood supply and have a negative effect on the function of the transplanted islet cells.
Borud et al. [10] argued that the fasciovascular flap is a good candidate for islet cell transplantation. We hypothesized that a denervated muscle flap, which has the abundant blood supply of a muscle without the negative effects due to contractile motion, could supply a suitable environment for islet cell transplantation. To the best of our knowledge, no studies have reported islet cell transplantation in muscle flaps. In this study, we aimed to evaluate the usefulness of muscle flaps for islet cell transplantation in diabetic rats by assessing insulin secretory capacity, glucose tolerance, and immunochemistry.
METHODS
Study animals and pancreatic excision
Approval for the study was obtained from the Institutional Review Board (No. H-0802-006-233), and the Institutional Animal Care and Use Committee (No. 08-244). The study was performed with isogenic Lewis rats (200–250 g). To obtain pancreases, midline incisions were made into the peritoneal cavity of 12 isogenic Lewis rats under isoflurane sedation. In each rat, two parts of the pancreatic duct, connecting to the duodenum and descending from the liver and gallbladder, were ligated. Ten milliliters of collagenase P fluid (collagenase 1 mg/mL; Roche, Mannheim, Germany) was injected into the common bile duct from the liver while checking for proper inflation. Thereafter, the pancreas was collected by separating it at the junction with the duodenum.
Islet cell separation
Each excised pancreas was placed in a 50-mL tube, which was then filled with collagenase fluid up to a total of 10 mL. The tubes were shaken 70 times/minute for 15 minutes in a 37°C water bath. Cold Hanks’ balanced salt solution (HBSS) fluid was added to the blocking enzyme, centrifuged at 1,600 rpm for 1 minute, and washed with 50 mL of HBSS fluid; this was repeated three times. After filtration through a 400- to 500-μm membrane, the collected pellets were added to cold HBSS and centrifuged at 1,600 rpm for 1 minute. These steps were repeated three times. The pellets were suspended by adding 12 mL of 1.10 g/mL Ficoll (Biocoll; Autogen Bioclear UK Ltd., Calne, UK). Thereafter, 7-mL volumes of four Ficoll concentrations (1.100, 1.096, 1.085, and 1.037 g/mL) were carefully added to create layers and then centrifuged at 1,800 rpm for 20 minutes to collect the islet cells in the 1.085 g/mL layer.
Pellets obtained after 1 minute of centrifugation at 1,300 rpm were washed in cold HBSS solution, and then centrifuged again in the same manner to remove viscous Ficoll. The pellets were then suspended in 20 mL of RPMI 1640 medium (Welgene, Gyeongsan, Korea) with the addition of 10% fetal bovine serum (FBS) and 1% antibiotic-antimycotic. They were then sampled four times with a 100-μL pipet. One sample was stained with dithizone; three samples were distributed into a six-well plate to count the number and size of the islet cells under a phase-contrast microscope (Fig. 1). The average number of cells were converted based on the sample volume to estimate the total number of extracted cells. From this, the amount of liquid corresponding to 3,000 islet cells was sampled and centrifuged at 1,300 rpm to collect the cells. RPMI (5 mM glucose+10% FBS+1% penicillin) was added to the pellet and placed in a 1-mL syringe for transplantation. At this step, the total volume did not exceed 0.2 mL.
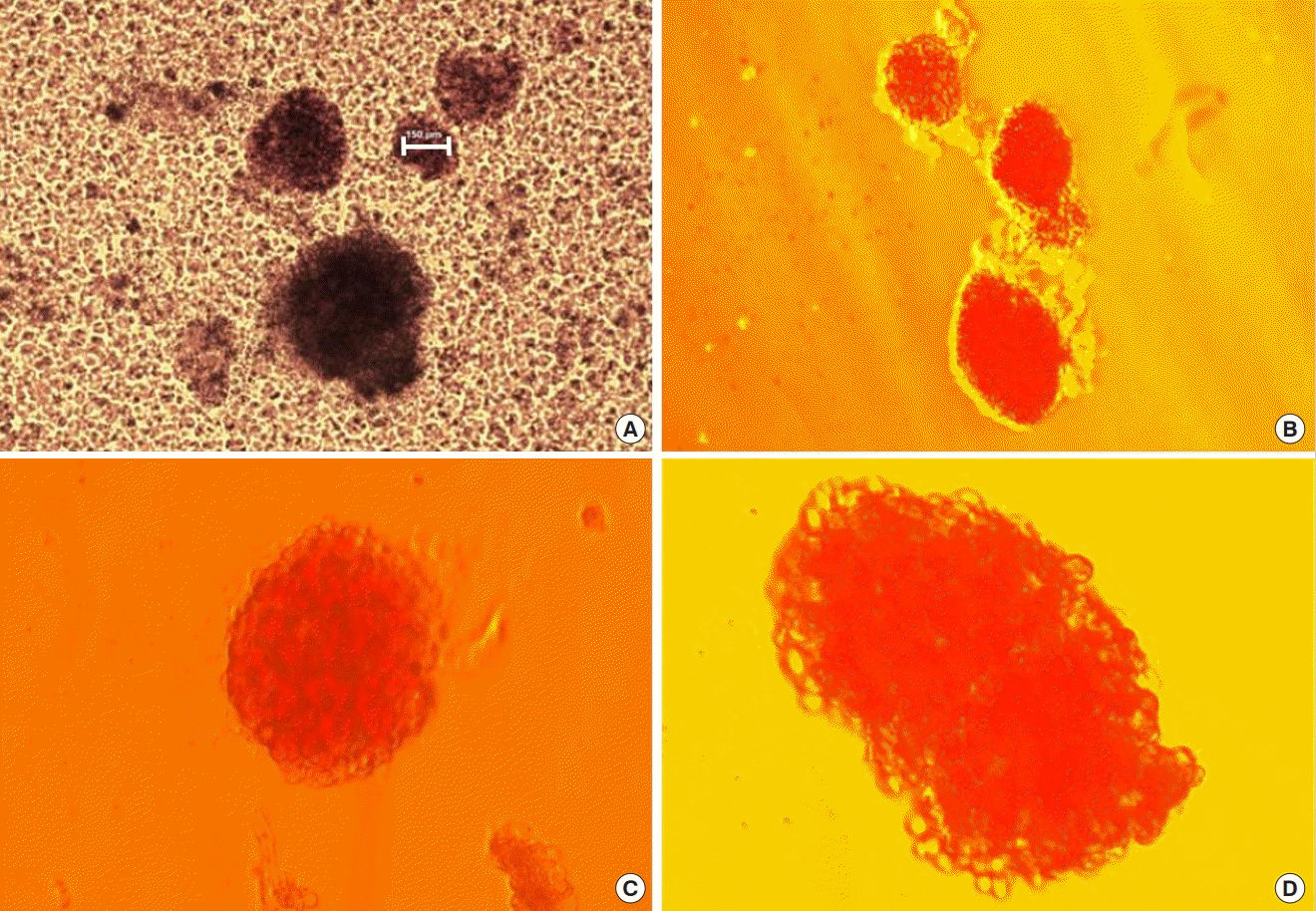
Microscopic view of isolated islet cells
Phase-contrast microscopic view of isolated islet cells before purification (A) and after purification and dithizone staining (B-D). In phase-contrast microscopy, the size of the islet is indicated with a white bar (150 μm). In dithizone staining, insulin-secreting β-cells are stained in red (A and B, ×40). Cluster of islets are observed (C and D, ×100). Islets are stained in bright red.
Islet cell transplantation
Twelve rats were injected with streptozotocin (Sigma Aldrich, St. Louis, MO, USA) into the abdominal cavity to induce diabetes mellitus (DM). The rats were judged to have DM when glucose levels in tail vein blood were maintained at >300 mg/dL for >7 days.
In group A (transplantation to the gastrocnemius muscle flap), after an incision was made in the hind limb under isoflurane anesthesia, the gastrocnemius muscle was identified, and the vascular pedicles (sural artery and vein) were preserved. After the nerves (gastrocnemius branch of the peroneal nerve) were sacrificed, the origin and insertion of the muscle were severed (Fig. 2); the proximal end of the muscle was then ligated to prevent leakage. After 3,000 islet cells were injected into the elevated gastrocnemius muscle flap using a 23-gauge syringe, the distal end of the muscle was ligated before closure (Fig. 3). After closure, a metal protector was placed to prevent self-mutilation.
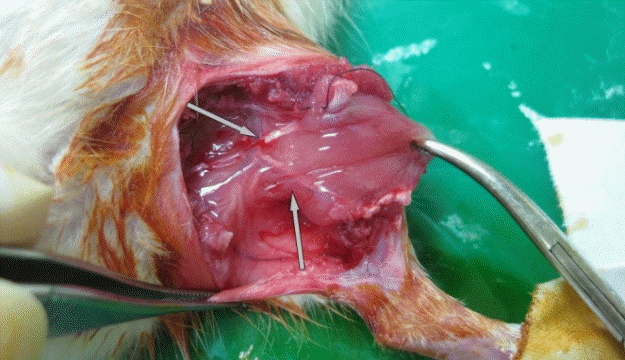
Elevation of a gastrocnemius muscle flap
After incising the thigh and calf skin, the muscle layers were also incised. The gracilis muscle was transected to ensure a better visual field. Vascular pedicles (gastrocnemius muscular branch of the sural artery and vein) are observed to enter the medial belly of the gastrocnemius muscle. The motor nerve innervating the medial belly of the gastrocnemius muscle (gastrocnemius muscular branch of the peroneal nerve) was sacrificed. The insertion of the muscle was detached. The cut end of the nerve was observed and the vascular pedicle supplying the gastrocnemius muscle was noted (gray arrows).
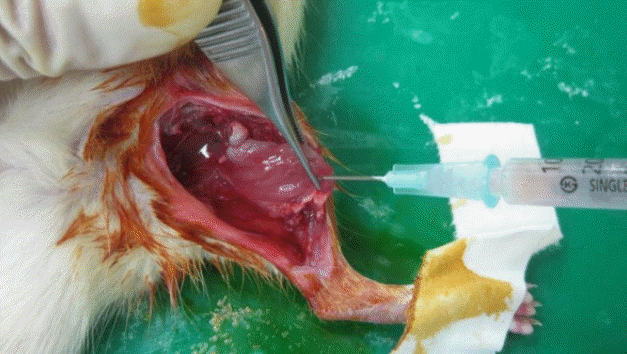
Injection of prepared islets into muscle flap
In total, 3,000 islet cells were injected into the muscle flap.
In group B (transplantation to the gastrocnemius muscle), the same incision was made under anesthesia, and 3,000 islet cells were injected into the gastrocnemius muscle.
Between 3 and 4 PM every day, blood glucose levels from the tail vein were determined and body weight was measured.
Glucose tolerance test
Four weeks after transplantation, glucose tolerance tests were performed in two normal rats and two DM-induced rats that had fasted for more than 6 hours from groups A and B. Glucose levels were measured before glucose loading and at 0, 5, 15, 30, 60, 90, and 120 minutes after loading.
Hematoxylin and eosin staining and immunostaining
Four weeks after transplantation, gastrocnemius muscles injected with islet cells were harvested from one randomly selected rat from each group. After paraffin-fixed tissues were sliced at a 4-μm thickness and placed on glass slides, they were processed in the usual manner for hematoxylin and eosin staining. For immunostaining, 3,3’-diaminobenzidine was utilized for color fixation, and hematoxylin was used for contrast.
Real-time reverse transcription polymerase chain reaction
Four weeks after transplantation, the muscle flaps were removed. Then, real-time reverse transcription polymerase chain reaction (RT-PCR) was performed for five gastrocnemius muscle flaps transplanted with islet cells, five gastrocnemius muscles transplanted with islet cells, five gastrocnemius muscles without transplantation, and five pancreases from normal rats.
After processing by pulverization, culturing, and centrifugation, the tissues were reacted in a 7300 real-time PCR system. Insulin I and II (Applied Biosystem, Waltham, MA, USA, Rn02121423_g1 and Rn01774648_g1) were used as primers. The acquired value was calculated using relative quantitation (RQ), with the standard pancreas sample as a value of 1.
Statistical analyses
Comparisons of the group with islet cells transplanted to the gastrocnemius muscle flap and the group with islet cells transplanted to the gastrocnemius muscle in terms of changes in blood glucose levels and body weight, as well as RT-PCR values, were analyzed using the Mann-Whitney U test, and glucose-loading test results were compared in the same manner. Within each group, comparisons of pre-transplantation levels and each period’s average level were analyzed using the Wilcoxon rank-sum test. For statistical analysis, SPSS version 26.0 (IBM Corp., Armonk, NY, USA) was used.
RESULTS
Transplantation of islet cells and alterations in blood glucose level/body weight
Group A consisted of six rats with 3,000 islet cells transplanted to the gastrocnemius muscle flap, while group B included six rats with 3,000 islet cells transplanted directly to the gastrocnemius muscle. One rat in group B died 15 days after transplantation. This animal was excluded from the data analysis because the reason for death was not apparent. The changes in blood glucose levels after transplantation of islet cells are shown in Fig. 4.
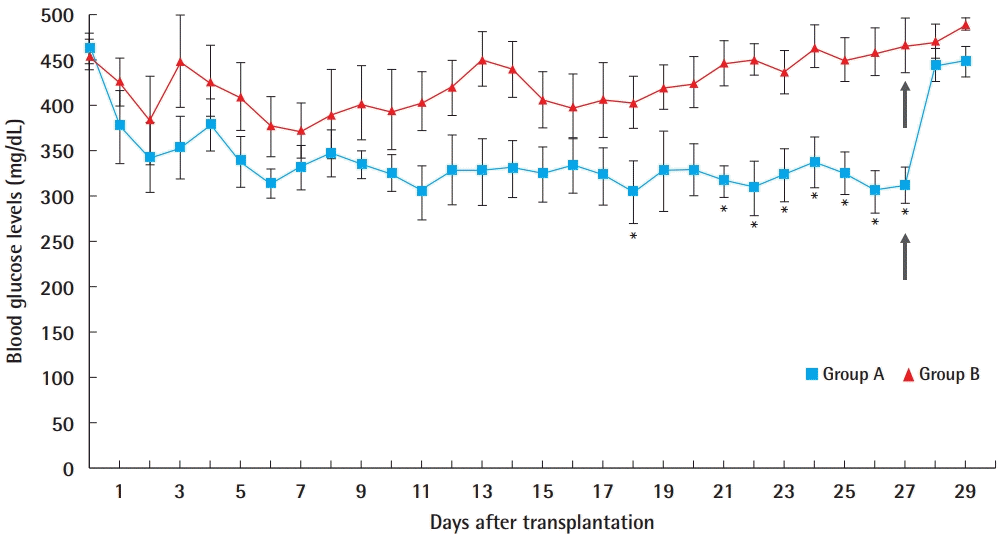
Blood glucose levels after transplantation
Average peripheral blood glucose levels at 4 PM (non-fasting glucose levels) after transplantation of 3,000 islet cells in muscle flap group (group A) and muscle group (group B). The gray arrow indicates the day of muscle flap removal. Significant differences are marked with asterisks (*).
In the comparison between the average values of the daily blood glucose levels from the two groups as analyzed by the Mann-Whitney U test, no statistically significant difference existed through day 17 (P>0.05). At day 18, the difference was significant (P=0.03). However, on days 19 and 20, the difference was not significant (P=0.52). After day 21, all P-values were significant.
To investigate whether a significant decline existed in blood glucose levels within each group, comparisons of values at pre-transplantation and on days 1, 3, 5, 7, 14, 21, and 28 were analyzed using the Wilcoxon rank-sum test. In group A, blood glucose levels decreased significantly from day 1 (Table 1). Moreover, blood glucose levels decreased immediately after transplantation, and a relatively constant glucose level was then maintained after day 7, but the values were not in the normoglycemic range. After flap removal on day 28, blood glucose levels abruptly increased.
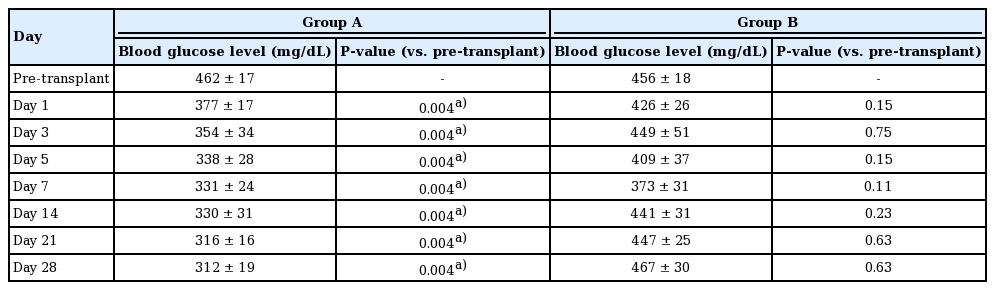
Blood glucose levels in each group over time in comparison to the pre-transplantation blood glucose levels
In group B, the average blood glucose level decreased in the early stages, although the decreases were not statistically significant, and then gradually increased (Table 1). As shown in the graph, blood glucose levels decreased until day 7, and then the levels increased and were maintained at higher levels than those in group A at all time points. After removal of the transplanted tissue, the blood glucose level in both groups increased and there was no significant difference between the two groups (P=0.662).
Changes in body weight after the transplantation of islet cells are shown in Fig. 5. The average daily body weight values of the two groups were compared using the Mann-Whitney test; there were no statistically significant differences during the entire time course (P>0.05).
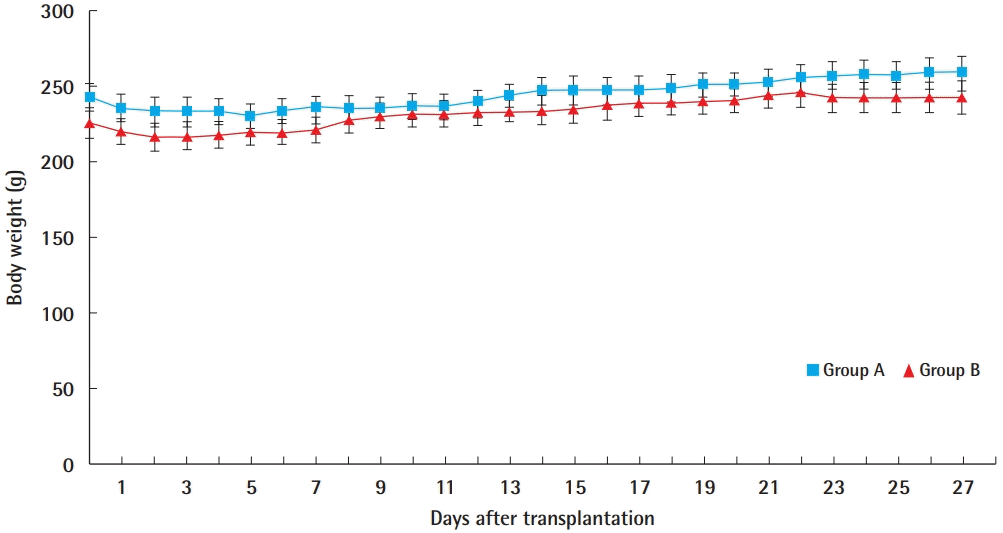
Body weight change after transplantation
Average body weight after transplantation of 3,000 islet cells in muscle flap group (group A) and muscle group (group B).
To investigate whether a significant decrease occurred in body weight within each group, comparisons of pre-transplantation body weight with the body weight measured at each time point were analyzed using the Wilcoxon rank-sum test (Table 2). In group A, body weight initially decreased 5 days postoperatively (P=0.046), but gradually increased starting on day 5 and recovered. In group B, no significant change was observed (P>0.05).
Glucose tolerance test
The results of the glucose tolerance test in each group are shown in Fig. 6. Unlike the non-fasting random glucose levels measured between 3 and 4 PM, fasting glucose levels showed nearnormoglycemic status in the group A (109±16 mg/dL). In group B, a hyperglycemic state was observed (186±54 mg/dL).
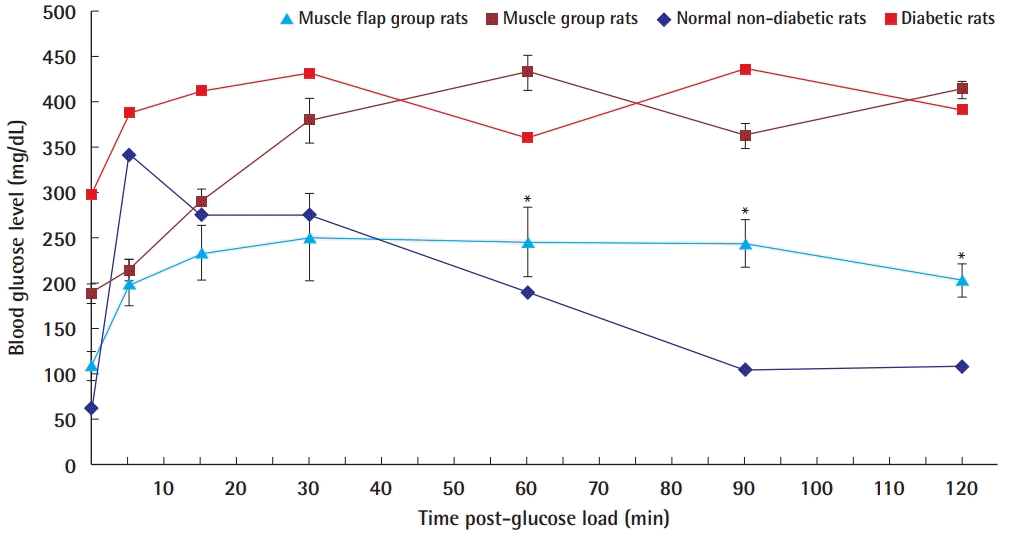
Glucose tolerance test 4 weeks after transplantation
Intraperitoneal glucose tolerance test (IPGTT) 4 weeks after transplantation of islet cells. The time points at which the glucose levels between the muscle flap group and muscle group showed significant differences are marked with asterisks (*).
Blood glucose levels in group A showed a statistically significant increase at 5 minutes (P=0.046) and reached a peak level at 30 minutes, which was a nonsignificant increase. Thereafter, glucose levels tended to decrease, although not significantly, but were significantly reduced from 90 to 120 minutes. In group B, blood glucose levels showed no significant decrease, as was seen in diabetic rats.
In the comparison of the blood glucose levels between the two groups using the Mann-Whitney test, no significant difference was observed from the pre-loading period to 30 minutes post-loading (pre-glucose load, P=0.476; 5 minutes, P=1.000; 15 minutes, P=0.257; 30 minutes, P=0.352), but were significantly different after 60 minutes (60 minutes, P=0.038; 90 minutes, P=0.010; 120 minutes, P=0.010).
Hematoxylin and eosin staining and immunostaining
The results of hematoxylin and eosin staining and immunohistochemical analysis at 4 weeks in groups A and B are shown in Figs. 7 and 8, respectively.
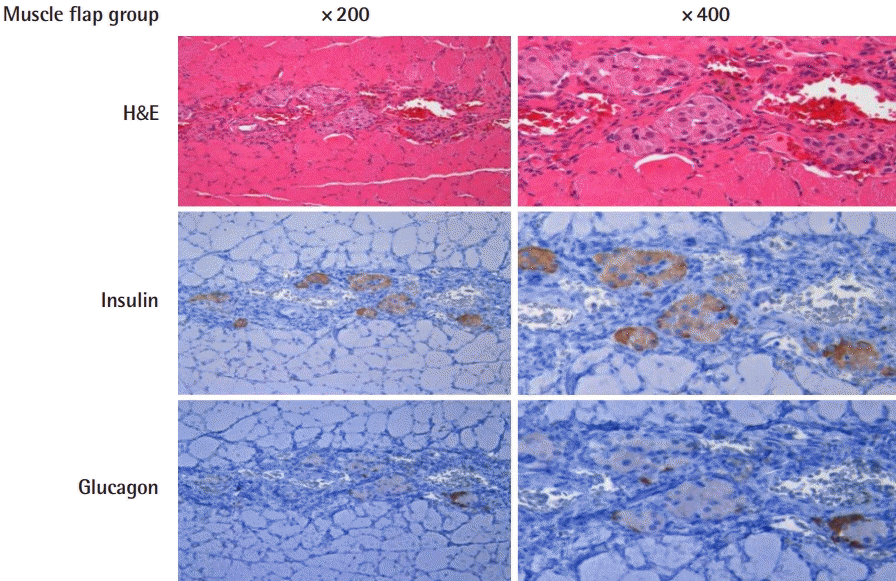
Immunohistochemistry of the muscle flap group
Hematoxylin and eosin staining and immunohistochemical staining of islet-transplanted muscle flap to anti-insulin and anti-glucagon antibodies.
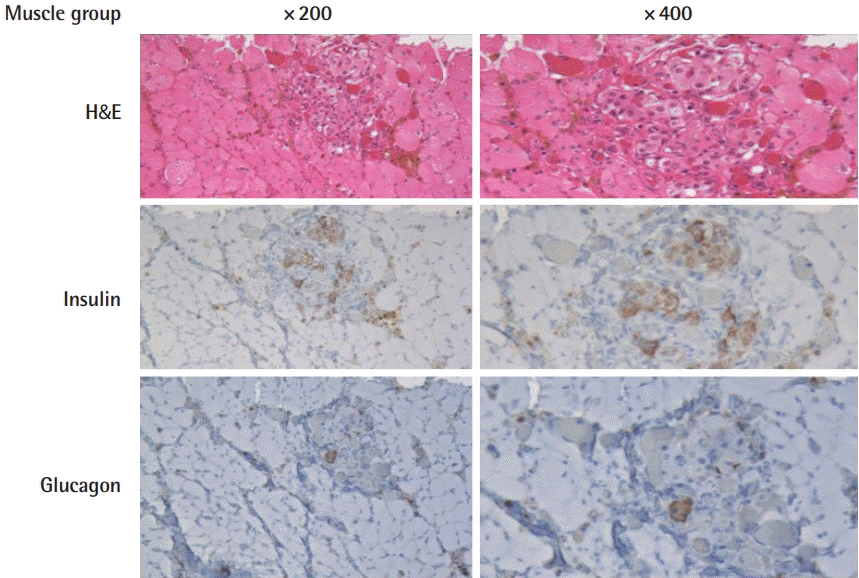
Immunohistochemistry of the muscle group
Hematoxylin and eosin staining and immunohistochemical staining of islet-transplanted muscle to anti-insulin and anti-glucagon antibodies.
Well-engrafted islet cells were observed in both groups with abundant granular cytoplasm and cell nesting, including one nucleolus each. Microvessels were well developed between the cell clusters, without fibrosis or inflammatory cell infiltration. Occasionally, features of old bleeds were evident, with hemosiderin pigmentation. More islet cells were found in group A than in group B, where the density of the insulin-positive beta cells was about 1.6 times higher in group A. This tendency was the same for individual islet cell clusters. Within islets in the normal pancreas specimens, glucagon-positive α-cells were identified in the peripheral areas, while insulin-positive β-cells were found in the central region. This distribution was also observed in the transplanted islets. Outside of the transplanted cell clusters, atrophied muscle fibers with angulated cut surfaces were observed, which was in concordance with the denervation-induced atrophy of muscle fibers.
Real-time RT-PCR
RT-PCR was performed for a quantitative comparison of the islet-transplanted muscle flaps, islet-transplanted muscles, gastrocnemius muscles without transplantation, and pancreas specimens from normal rats. The results are shown in Fig. 9.
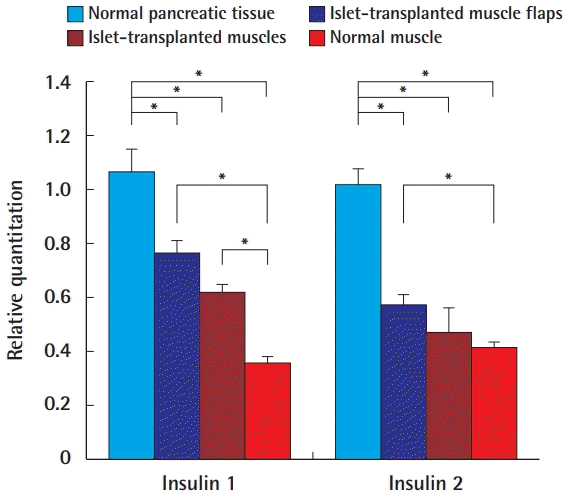
Real-time reverse transcription polymerase chain reaction results
Real-time reverse transcription polymerase chain reaction results in normal pancreatic tissue, islet-transplanted muscle flaps, islettransplanted muscles, and normal muscles. Significant differences are marked with asterisks (*).
The RQ values of both insulin 1 (0.762±0.050) and insulin 2 (0.571±0.037) were significantly lower in group A than in the normal pancreas group (P=0.009, insulin 1, 1.066±0.081; P= 0.002, insulin 2, 1.017±0.060), but significantly higher than the RQ values of the normal muscle group (P=0.002 and P=0.009, respectively). The RQ values of both insulin 1 (0.619±0.026) and insulin 2 (0.472±0.093) were significantly lower in group B than in the normal pancreas (P=0.004 and P=0.004, respectively). However, compared with the normal group, the RQ value of insulin 1 was significantly higher (P=0.004), and there was no significant difference in the levels of insulin 2 (P= 0.329). The RQ values of both insulin 1 and 2 were higher in group A, but the difference between the groups was not statistically significant (P=0.082 and P=0.429, respectively).
DISCUSSION
Transplanted islet cells need sufficient oxygen and nutrients to survive, as reflected in the selection of recipient sites by many researchers. Most organs, including the liver, spleen, and omentum, have well-developed blood vessels and rich blood supplies [11,12]. In the kidneys, the parenchyma is well developed and has a high blood supply, but this not the case in the kidney capsule. According to Carlsson et al. [2], the kidney membrane has an oxygen partial pressure of about 15 mmHg, nearly half of that in the kidney parenchyma, which is very low considering that the average oxygen partial pressure of the pancreas is 30–40 mmHg. The oxygen partial pressure in the latissimus dorsi muscles of rabbits is known to be approximately 20 mmHg [13]. Although these values are not for rats, making a direct comparison difficult, these findings suggest a tendency for oxygen pressures to be higher in the muscles than in the kidney membrane. Furthermore, the well-developed capillary networks of muscles are advantageous as the transplanted cells will be closer to blood vessels. In our results, many blood vessels were observed around the living cells.
Although some papers reported normalization of glucose levels after islet cell transplantation into the muscle, it is difficult to ascertain whether the muscle was more successful than the hepatic parenchyma or the renal capsule. Rafael et al. [14] failed to induce blood glucose normalization by implanting 160,000 islet equivalent cells into the brachioradialis muscle of a 7-year-old girl who underwent pancreatectomy for hereditary pancreatitis. Although normalization was not achieved, it was possible to reduce the insulin requirement. Moreover, the insulin concentration in the blood from the transplantation-side arm was higher than that in the other arm. Stegall [15] reported islet cell survival in two patients in biopsies performed after transplanting subtherapeutic numbers of islet cells into the forearm muscles of three patients with diabetes who underwent kidney transplants. Other experimental models on animals have shown that the muscle is a suitable site for islet cell transplantation [6,7]. Notably, however, all sites were skeletal muscles that contract and relax. This movement could interfere with the process in which the transplanted cells stay physically steady for re-vascularization. Moreover, the compressed vessels during muscle contraction are disadvantageous for appropriate blood supply. In the muscle flaps in this study, the researchers presumed that perfusion could be maintained adequately and that a static environment could be created if the nerves are blocked and muscle contraction is prevented. Furthermore, because of the shortened muscle length and reduced tension after detachment, the muscle cannot contract. Therefore, the environment of the denervated muscle flap is more advantageous for the engraftment of islet cells.
In transplanting liver cells through the portal vein, some islet cells undergo an immediate blood-mediated immune reaction (IBMIR), which is believed to occur because of the proteins expressed on the cell surface. This causes a coagulation cascade, in which the complement system is activated in clotted blood and leukocyte infiltration leads to cell destruction [1]. Therefore, a significant amount of cell loss occurs in the early stage of transplantation, and attempts have been made to reduce it.
Moberg et al. [16] reported that nicotinamide could regulate the expression of tissue factors in islet cells. Attempts have been made to coat the cell surface with heparin to block the clotting process [17]. Some researchers used dextran as an antiplatelet agent [18]. However, it is impossible to entirely prevent IBMIR because direct blood contact cannot be avoided in the portal vein. Bennet et al. [19] found that destruction of the islet cells by inflammatory cells was observed upon mixing whole human blood cells, even with blood type concordance. When heparin and soluble complement receptor 1 were added, destruction of the islet cells was reduced. However, transplantation into muscle minimizes the initial cell loss by IBMIR because direct blood contact is avoided. Moreover, cell leakage to the systemic circulation can be limited. In the present study, although some hemosiderin pigment was observed due to slight bleeding, the amount was not significant.
The successful graft of endocrine tissues ensures appropriate hormone release and function in response to specific signals. For example, surgically resected parathyroid glands are known to perform their regular function when transplanted into an appropriate site. For the parathyroid, transplantation into muscle has been widely used as long-term follow-up has shown excellent results [20]. This indicates that the response and release function of endocrine tissue is maintained when implanted into the muscle.
Under normal circumstances, insulin secreted by the pancreas is transmitted through the portal vein to the liver, which is known as the primary organ of action for insulin. It can be considered physiologically valid that the insulin secreted from the transplanted cells is transferred to the liver through the portal circulatory system. De Vos et al. [21] observed changes in blood glucose in two groups of rats when injected into the portal vein and in another vein, with various concentrations of insulin. In their study, there was no difference between the two groups when insulin was injected at low concentrations, but when high concentrations of insulin were injected in the portal vein, less hypoglycemia was observed and glucose levels recovered to normal. Thus, transplantation through the portal vein might be more advantageous because insulin acts directly, quickly, and sensitively. Similarly, in transplantation into muscle, if the islet cells transplanted into a free muscle flap are transferred close to a portal system, their function could be appropriately induced and insulin would be secreted well in response to blood glucose concentrations.
In our experiment, the transplanted islet cells successfully survived both in the muscle flap and muscle groups, as shown in the immunohistochemical results. The density of engrafted islet cells was higher in group A, implying that the muscle flap is a better environment for islet cell survival. At 21 days after transplantation, the blood glucose levels in group A began to show significantly lower values than those in group B, implying better insulin secretory function. However, measurements of blood glucose levels of diabetes-induced rats not receiving islet cell transplantation would have provided information on baseline glucose variation, allowing a better comparison to be made.
Body weight did not show significant differences between the two groups. The initial body weight decrease in group A was probably due to postoperative stress. Though not statistically significant, an initial body weight decrease was also observed in group B.
In the glucose tolerance test, the curve of group B showed a similar tendency to that of diabetic rats. However, group A showed a tendency somewhere between normoglycemic and hyperglycemic rats. This indicates that group A significantly gained insulin secretory function compared to group B, but not good enough to achieve normoglycemia. We believe that if we had given more time for the islet cells to adapt, then the curves would have shown trends closer to those of normoglycemic rats.
The trends shown in the real-time RT-PCR results were also consistent with the glucose tolerance test results. Although the difference between groups A and B was not significant, their respective positions inside the spectrum of normoglycemia to hyperglycemia were quite apparent. We believe that a larger sample size would have yielded statistical significance.
Integrating our results, we find it reasonable to argue that the denervated muscle flap is a better vehicle for islet cell transplantation than the muscle.
There have been no reports of islet cell transplantation using muscle flaps with microvascular anastomosis. However, in Lewis rats, islet cell transplantation into a fascia-fat-free flap has been reported. Blood glucose levels were lowered by free fascia-fat flap transfer in the same type of diabetes-induced rats [22]. This report suggests that the muscle flap has the potential to be used at other sites or in other individuals through free flap transfer. Using a free flap for islet cell transplantation has another potential benefit of being possible to combine with simultaneous reconstruction of a diabetic foot or any other defect.
The gastrocnemius flap was the first muscle flap established in rats [23]. A detailed study of its anatomical structure was reported by Tonken et al. [24]. In the transplantation experiment, the gastrocnemius muscle had a sufficient muscle volume to allow cell transplantation and a simple vascular structure facilitating the approach. It is easy to perform this neurosurgical procedure, and there is a long vascular diameter. However, the gastrocnemius flap has the drawback of causing functional problems in the lower limbs. Therefore, in clinical practice, it is recommended to use latissimus dorsi, gracilis, or rectus abdominis flaps with the perforator technique.
This study has several limitations, such as a small sample size and short follow-up period. Moreover, inclusion of a negative control group would have provided information on baseline glucose variation, allowing a better comparison. However, we believe that our results are sufficient to conclude that denervated muscle flaps are a promising potential vehicle for pancreatic islet cell transplantation.
Notes
Conflict of interest
No potential conflict of interest relevant to this article was reported.
Ethical approval
The study was approved by the Institutional Review Board of Seoul National University Hospital (IRB No. H-0802-006-233) and the Institutional Animal Care and Use Committee of Seoul National University Hospital (IACUC No. 08-244) and performed in accordance with the principles of the Declaration of Helsinki.
Author contribution
Conceptualization: BK Kim. Data curation: JL Park, T Kim, BK Kim. Formal analysis: BK Kim. Methodology: JL Park, BK Kim. Project administration: BK Kim. Visualization: BK Kim. Writing - original draft: JL Park, T Kim, BK Kim. Writing - review & editing: JL Park, T Kim, BK Kim.